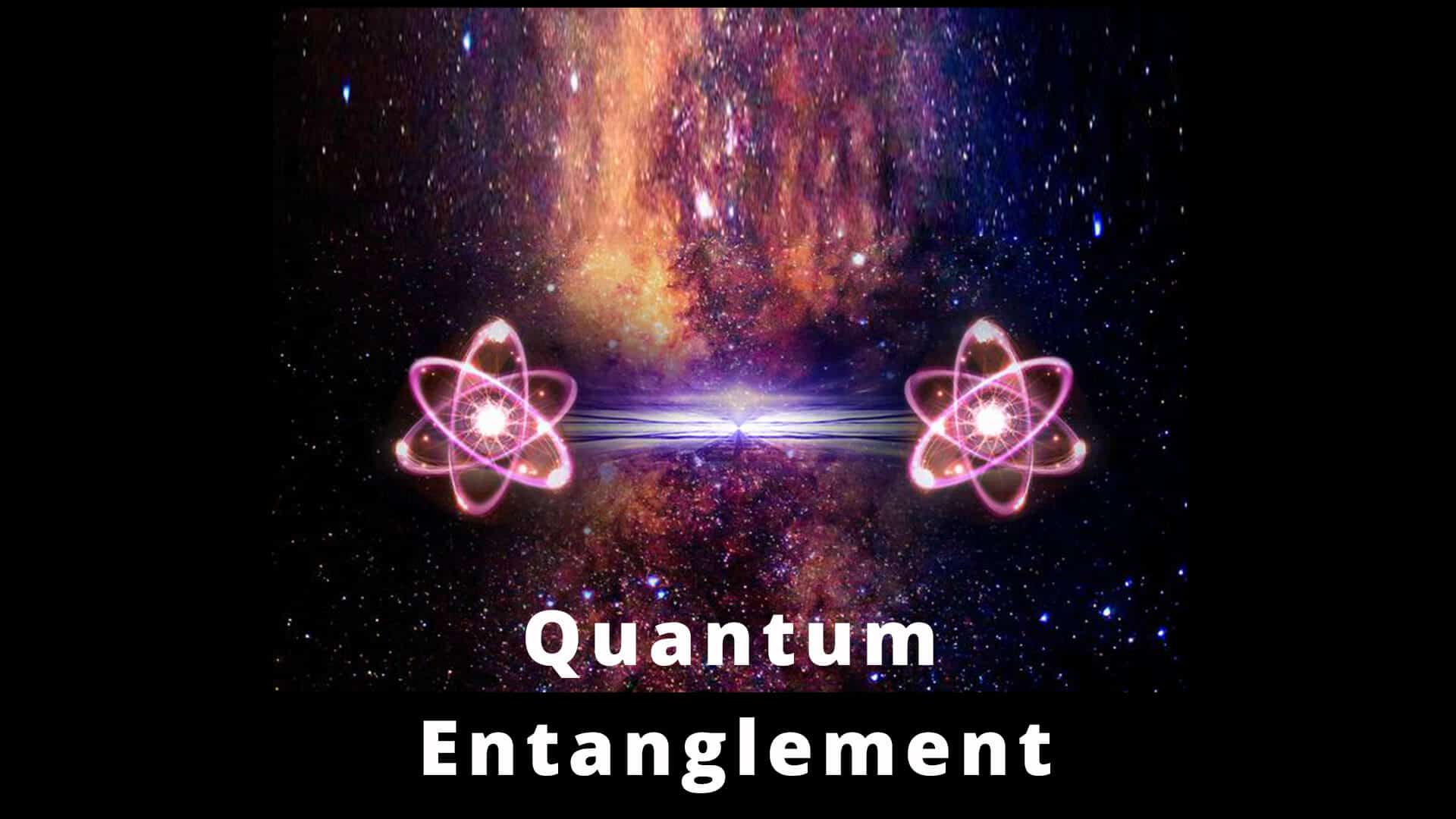
What is Quantum Entanglement?
Quantum Entanglement
Entanglement is perhaps the most interesting property that distinguishes the quantum world from the classical one. It underlies the proof of the fundamental uncertainty of events in the quantum world, and modern quantum technologies such as quantum communication, quantum computing and ultra-precise quantum instrumentation are based on it.
Just the other day, American physicists invented an ultra-precise atomic clock based on quantum-entangled atoms, and a group of Chinese scientists demonstrated an optical quantum computer that works many times faster than the fastest classical supercomputer.
We are going to talk about quantum entanglement. You’ve certainly heard of this strange expression and the wacky consequences it had such as being able to teleport objects … without really knowing what quantum entanglement is .
The concept of “entanglement” was introduced by Erwin Schrödinger in 1935 – the same theoretical physicist who “tortured” an imaginary cat. However, the concept entered widespread use only in the 1990s, with the appearance of the first quantum communication systems and prototypes of quantum computers.
Schrödinger’s cat experiment is an example of an entangled system. The cat is in a box and in that box is a deadly gas locked in a vial that will only break under certain conditions. These conditions are determined by a small radioactive sample.
If the atoms disintegrate, then the flask breaks and the cat dies. Not having opened the box, the cat is both dead and alive, and we can know if it is dead or alive without opening the box simply by observing the state of the radioactive sample.
Particles are characterized by what are called “quantum numbers”. These can be: the electric charge, the energy of the particle, the spin – quantum angular momentum – and many others.
When two particles are said to be entangled, the state of the first particle is determined with certainty by the state of the second particle.
By state I mean the quantum numbers of these particles or their characteristics. This means that if we have a system of two entangled particles and measure the characteristics of the first particle, we will know the characteristics of the second particle with certainty.
But you know that a basic principle of quantum mechanics is never to know with certainty the quantum state of a particle and that this is only determined by the experimental measurement of the system. This principle is violated when the system is entangled.
Consider an analogy of quantum entanglement in everyday life with two billiard balls. Both are characterized by their position and speed. At the start, the balls form two distinct and non-entangled systems. If one ball hits the second – at a known angle – then the system becomes entangled because if we study the position and speed of a ball, we immediately know the position and speed of the second ball. The system is then said to be entangled and we can consider the two balls as a single particle.
In quantum mechanics it’s pretty much the same thing. Suppose we have two particles in contact at time t. They can be characterized by what is called in quantum mechanics a wave function which takes into account the two particles. When these particles separate, they can be characterized independently of one another by two respective wave functions.
This means that there are three wave functions to characterize the system, one of which links the first particle to the second. This is why if we know the wave function of the first particle, we instantly know the wave function of the second particle.
The following problem then arises: does this mean that information can travel at a speed greater than that of light?
Well not at all. The observation of one of the two particles results in the disappearance of the entangled state of the system. Why?
Simply because it is impossible to observe a particle without influencing it, in order to observe these particles, physicists are obliged to make them interact with other particles so as to make the phenomenon macroscopic and therefore analyzable.
This means that in reality, it is impossible to instantly know the state of the second particle so that the information cannot travel at a speed greater than that of light.
There are many consequences to this phenomenon. Many concepts derive from this science and appear to have physical reality but are by no means demonstrated. These are hypotheses that I want to present to you and you can choose to believe them or not, the point is simply to create the debate.
Quantum entanglement would allow objects to be teleported. This is a phenomenon that I will develop a little more in a future article.
Another implication of quantum entanglement is of a more spiritual nature and is to say that the universe is a single entity and that we are a part of that entity and any event that takes place in the universe affects us and vice versa.
Key facts of quantum entanglement:
- Entanglement is a quantum phenomenon in which at least two particles share the same properties. When we measure the state of one of them, the other entangled particles instantly take on the same value, regardless of the distance between them.
- Bohr and Einstein were opposed on the interpretation to be given to the phenomenon. Niels Bohr, one of the founders of quantum mechanics, considered the probabilistic nature of the predictions of quantum mechanics to be fundamental. For Einstein, this probabilistic character is the consequence of an incomplete theory and that there are hidden variables to be discovered. This is called the EPR paradox (named after its authors Einstein, Podolsk and Rosen).
- John Bell formulates in 1964 mathematical inequalities respected by a possible theory with hidden variables but violated by quantum mechanics. Aspect’s experiments at Orsay helped settle the issue in favor of Bohr’s vision.
- Entanglement serves as a basis for the development of new applied technologies such as quantum computers and simulators, quantum cryptography and quantum teleportation.
Definition of quantum entanglement
Let’s first explain what an entangled state is. To begin with, quantum entanglement is always a story about a two-particle system- one atom or photon cannot be entangled. The system can be multiparticle, but then, to determine its entanglement, you still need to divide it into two subsystems and consider their correlations.
For the particles to be entangled, they had to interact sometime. If they have never interacted, then they are not confused. As an example: two particles were formed as a result of the decay of one particle.
But then they do not physically interact, there are no forces between them. It’s just that their state is such that they show correlations in different dimensions that cannot be described from the point of view of classical physics.
Is it mathematically expressed in some kind of equation?
Quantum states are described by a wave function. Accordingly, if we have two systems, then they are described by the joint wave function Ψ ( x 1, x 2), which depends on the parameters of the first system ( x 1) and the second system ( x 2).
And if this joint wave function cannot be represented as a product of the wave functions of its subsystems, then such a state is called entangled. Physically, this means that the parameters of these systems are related to each other.
And if I measure a parameter of one system, then I immediately receive information about the parameter of another system. It is important to distinguish entangled states from states exhibiting classical correlations.
Let me give you a simple example of classical correlations. We have a drunken shooter with a double-barreled gun. It fires randomly in all directions.
And it is clear that every bullet – no matter what barrel it came from – can hit in any direction.
But since the shooter simultaneously releases two bullets, then where one bullet went, the other will go about the same. These bullets are more or less connected to each other, and if I measure the coordinates of one, I can roughly understand where the other hit.
Another example of classic correlation. I have a pair of boots out of the same box. I randomly put one in one box, the other in another. One of my colleague go on Mars, the other to Venus. They receive them, open the boxes and see: “Aha, I have a left boot, so he has a right one.” And vice versa: “Yeah, I have a right one, so he has a left one!” That is, by measuring the state of a boot in their box, they can understand the state of a boot from a colleague on another planet.
What are the nonclassical correlations that manifest themselves in entanglement?
Imagine that we with boots can carry out some other type of measurement, which in the same way – for the right, that for the left – would give different results with equal probability.
For example, I can take a boot, toss it and watch where it falls: to the right or to the left – that’s how they used to guess. And now my experimenters on Mars and Venus also throw up their boots, and if their boots fall in the same direction, it turns out that the results of their measurements are related regardless of what type of measurements they make. These non-classical correlations are called entanglement.
How can quantum entanglement be practically applied?
If we talk about the applied applications of entanglement, then these are quantum metrology, quantum communication and quantum computing. In various metrological applications, the use of entangled particles allows for more accurate measurements of time, distance, electric and gravitational fields, etc.
Entanglement is a resource in quantum computing. The point is that if we have a multiparticle (for example, multiphoton) system, then in the general case the state of such a system will be confused. It turns out that a lot of information is needed to describe such a state.
If I increase the number of quantum bits, my number of coefficients will grow as 2n. i.e., this is a very fast exponential growth. (Now, in connection with the covid pandemic, we have all learned what an exponential growth is, when the number of infected doubles every two or three weeks.) Thus, if I have at least 50-60 quantum bits, then no computer will be enough for me, to record their condition there.
If I cannot calculate a quantum system, then I can do something with this system that a regular computer cannot. This is at the heart of quantum computing. Now various quantum computers are being developed – so far they have a rather limited number of quantum bits, a few dozen, but when there are several hundred or thousands of them, then they will already be systems on which it will be possible to solve a certain range of important problems.
Earlier, a group of Chinese scientists demonstrated a quantum simulator that had a hundred-channel interferometer, and there were such tricky squeezed states of light (states that contain only an even number of photons) at the input.
Scientists have measured the number of photons at its outputs and have shown that it is impossible to obtain similar results using a conventional computer. Even for a supercomputer it will take tens and hundreds of years. This is what is called quantum superiority.
For the first time, a similar experiment demonstrating quantum superiority was carried out by Google, they did not use photons, but superconducting qubits.
Here, of course, you need to understand that while such a quantum simulator solves only one problem – it simulates itself. But this is the first step towards quantum simulators that can solve the problems of quantum chemistry, logistics and other useful problems.
Have there been examples of successful quantum communication at a distance?
The most ambitious experiment of this kind was staged several years ago by the Chinese. They distributed pairs of entangled photons from the satellite: one photon flew to one city, the other to another city.
The distance between the cities was over 1000 km. In these two cities, measurements were made on these photons, and the results were correlated, they could be used further to encode information and transmit secret messages.
But this is, of course, a demonstration experiment. And commercially available systems of quantum cryptography today are limited to a range of about 100 km.
Related Articales
Recently Posted
-
भगवान गौतम बुद्ध जीवन परिचय | Gautam Buddha in Hindi
December 15, 2022. -
कार्बन के अपररूप Allotropes of Carbon in Hindi
November 5, 2022. -
मिश्र धातु किसे कहते हैं? उपयोग, नाम, गुण Alloy in Hindi
July 27, 2022. -
गलनांक किसे कहते हैं? परिभाषा, उदाहरण Melting Point in Hindi
July 20, 2022. -
परिमाप किसे कहते हैं? Perimeter in Hindi
July 19, 2022.